Nature has published a paper by the MICE collaboration, in which they demonstrate a technique that could carry the search for new phenomena in lepton-antilepton collisions to extremely high energy and provide uniquely well-characterized neutrino beams.
We’ve made great progress colliding beams of high-energy particles; we’ve gained a better understanding of matter and its interactions, refined the standard model and, in expanding the applications, we can analyze chemical structures or even treat cancers.
This has predominantly been done using beams of stable particles; protons, anti-protons, electrons and positrons, but there’s potential for using muon-antimuon collisions to produce beam energies that would dwarf the best of our current efforts.
What makes a Muon different?
Almost identical to electrons, muons are fundamental particles, but they’re around 200 times heavier than an electron and unstable too. It’s this extra mass that allows for higher energies and if muons were stable, they would be the perfect particle to accelerate!
When accelerating particles, you also need to take synchrotron radiation into account; the radiation is given off when a charged particle is accelerated perpendicular to its velocity. This radiation takes the form of photons being emitted (so energy is lost) and since most particle accelerators are circular, it can massively take away from the energy that ends up going into the final collision*. Synchrotron radiation increases on a scale of 1/m4; so electrons will emit more than their heavier brother the muon, but the proton will emit the least. This means we can keep building more and more powerful proton accelerators, but since they’re not fundamental particles the collisions are much “messier”.
*n.b Synchrotron Radiation isn't always a problem! For example, the Diamond Light Source creates synchrotron light to be used for a range of experiments.
There's also the advantage that the Higgs coupling is proportional to the mass of the muon. In the Standard Model, fundamental particles (electrons, muons, quarks, and short-lived particles like the Z boson) are massless. The Higgs particle gives them mass by how strongly it couples to them: light particles have low couplings and heavy particles have high couplings. The masses of the particles seem to be quite random with a range of values - electrons are over 300,000 times lighter than the heaviest known particle, the top quark.
As the muon mass is proportional to the Higgs Coupling, the chances of directly producing a Higgs boson at a muon collider are much higher - around 200 times more than with electrons. What's more, the theoretical description of muon decay is so precise that the beams of neutrinos created when muons decay can be used to try and understand aspects of the big bang and the beginning of our universe – in particular, why there’s so much more matter than anti-matter left behind.
However, for us to avoid using muons for so long, you can imagine there are numerous difficulties they come with; for example, muons have a lifetime of only 2.2μs, during which time they have to be captured and accelerated.
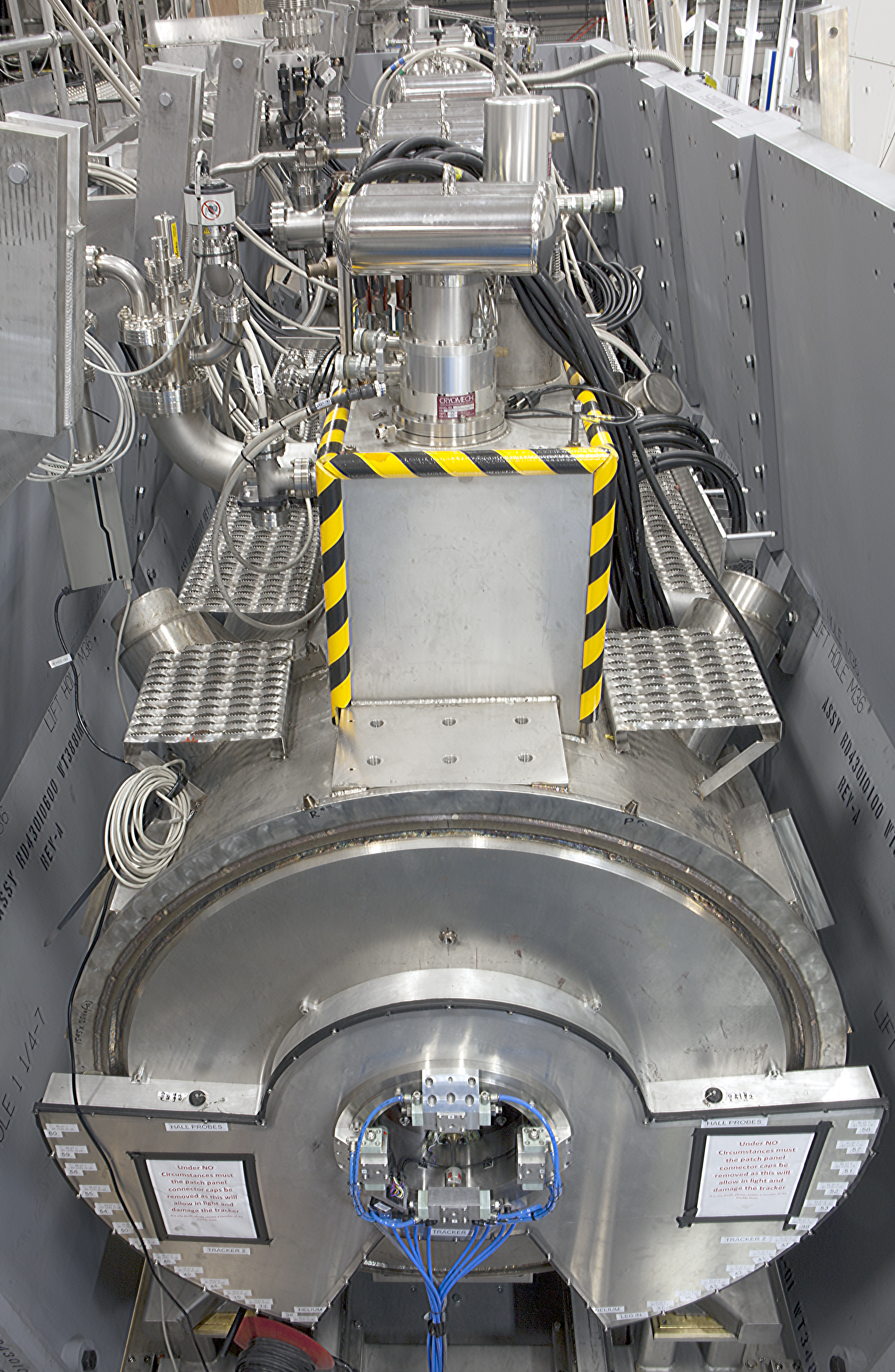
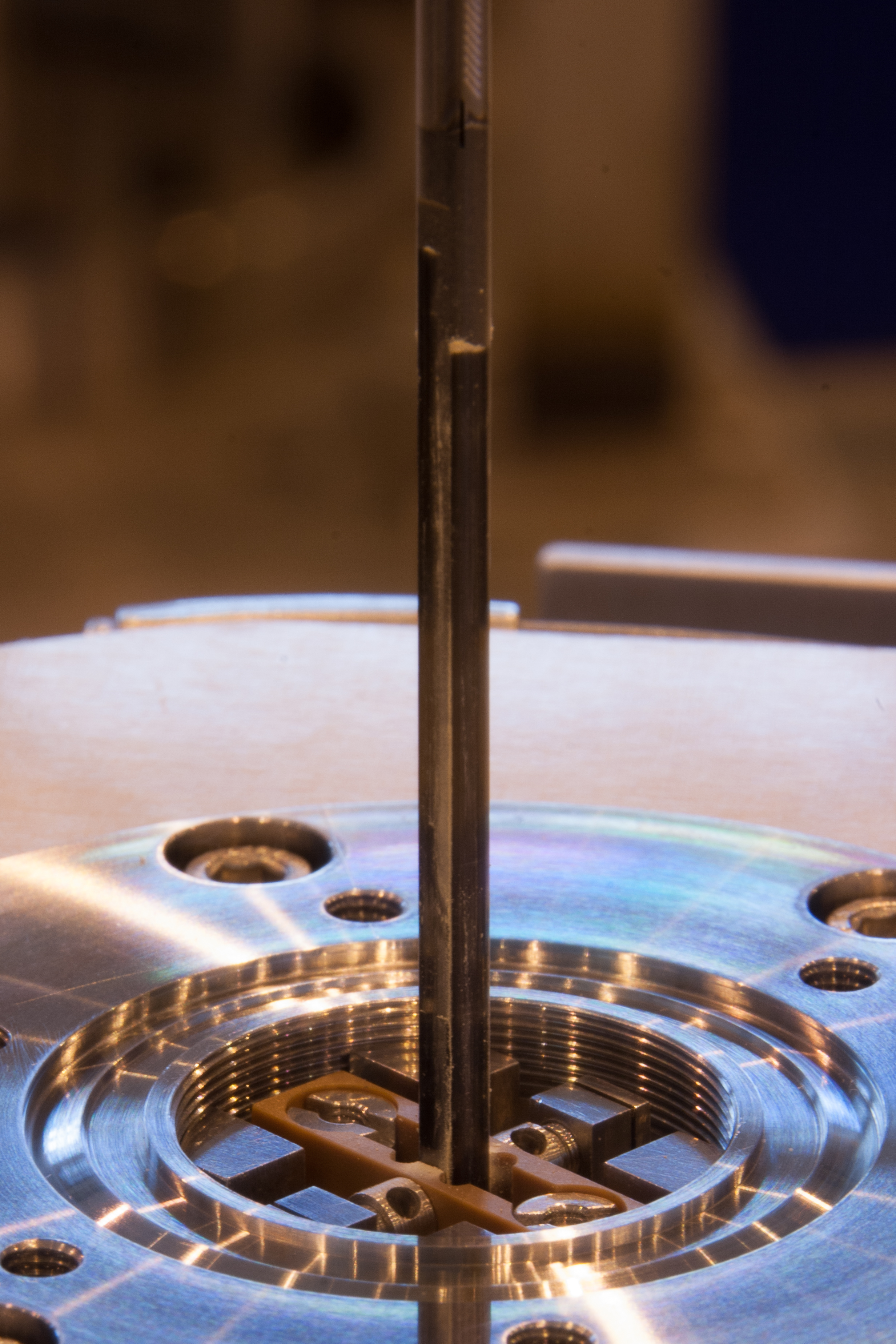
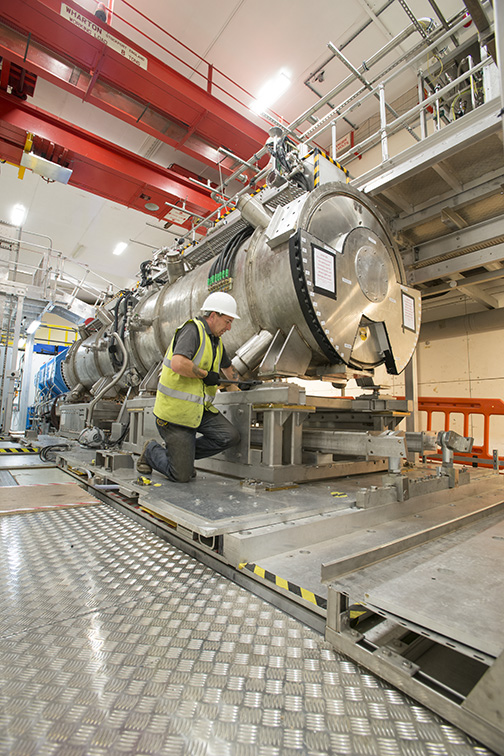
The problem with Muons...
The main breakthrough made by the MICE (Muon Ionization Cooling Experiment) collaboration is in overcoming difficulties arising from the way in which muons are produced. When, for example, protons are created(from separating hydrogen atoms/ions), they all head in the same direction, rather like a military marching band. Muons, on the other hand, form more of a ‘cloud’ and can be likened to a mixed group of pedestrians crossing a bridge; moving side to side, some distracted by the sights, as they travel.
If we used a cloud of muons as is, we would get a beam that is 10s of centimetres in diameter. The magnets needed for an accelerator that could accept this would be expensive both to build and run. Furthermore,
the particle intensity would be too low and so the chances of them
colliding and producing interesting physics are also low.
...And how to fix it
The
process of reducing the beam diameter, making this cloud less diffuse and getting the muons moving in the same
direction is called cooling. “Ionization cooling” was first proposed in
the 1970s and in the early 1980s Simon Van Der Meer solved a similar problem
for anti-protons; called “stochastic cooling” and it earned him a Nobel
Prize. The problem is that stochastic cooling takes a few seconds, but muons
live for a few millionths of a second. In the 1990s ionization cooling
became a theoretically operable technique but putting it into practice
was still formidable.
The
MICE collaboration was formed in the early 2000s and developed the
novel technique shown below, which has now been proven to work.
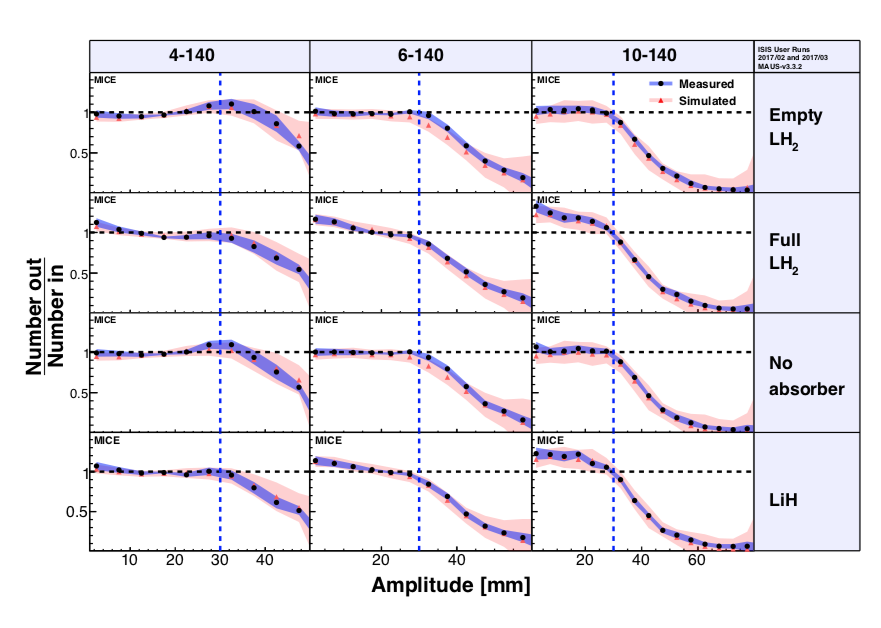
The figure above shows the downstream to the upstream ratio of a number of events. A ratio greater than unity in the beam core
is evidence for ionization cooling and is evident for 6-140 and 10-140 beams with both the full LH2 absorber
and the LiH absorber. The effect predicted from the simulation is shown in red, while that measured is shown in
black. Taken from https://arxiv.org/pdf/1907.08562.pdf
Making the muon beam
The experiment took place in the UK using the MICE muon beam-line, part of the ISIS neutron and muon source. Fast-moving protons strike a target (such as tungsten or mercury) which produces debris of pions, kaons and muons. The pions produced are separated and directed a long a series of magnetic lenses where they quickly decay to produce extra muons (and neutrinos). Muons are then captured by beamline elements to form a third beam. - And all of this must be done in under 2.2μs, the lifetime of a muon.
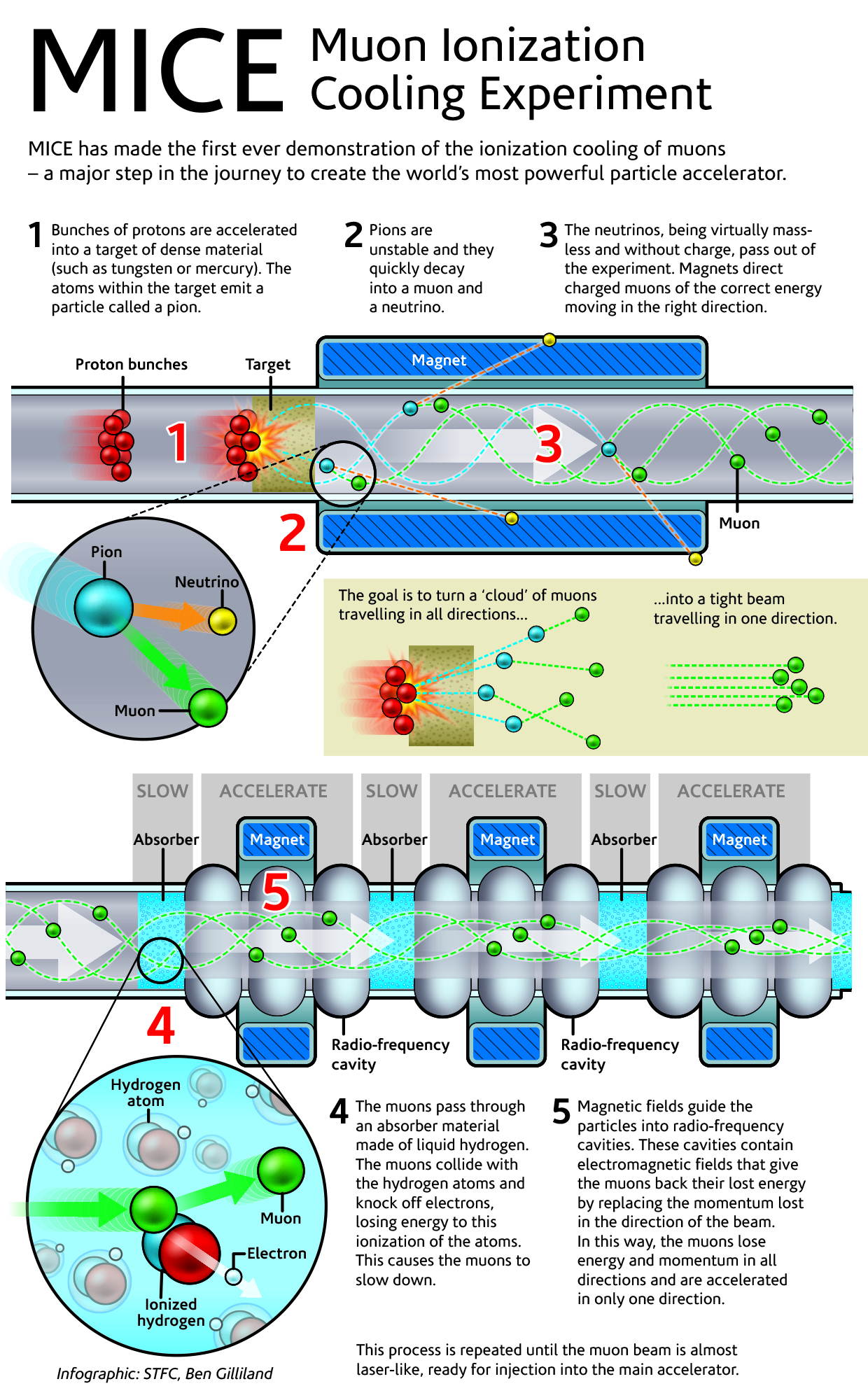
LINKS FOR MORE INFORMATION:
MICE Collaboration home page
MICE's most up-to-date page (as of 06/02/2020) for the public
Our MICE page
MICE Infographic
STFC article following MICE's publication in Nature
CERN Courier article on MICE
Quotes from members of the collaboration
“The enthusiasm, dedication, and hard work of the
international collaboration and the outstanding support of laboratory personnel
at STFC and from institutes across the world have made this game-changing
breakthrough possible,” commented Professor Ken Long from Imperial College
London, spokesperson for the experiment.
Dr Chris Rogers, based at ISIS and the collaboration’s Physics
Coordinator, explained: “MICE has demonstrated a completely new way of
squeezing a particle beam into a smaller volume. This technique is necessary
for making a successful muon collider, which could outperform even the LHC.”
Professor Alain Blondel, spokesperson of MICE from 2001 to
2013, and Emeritus Professor at the University of Geneva commented: “We
started MICE studies in 2000 with great enthusiasm and a strong team from all
continents. It is a great pride to see
the demonstration achieved, just at a time when it becomes evident to many new
people that we must include muon machines in the future of particle
physics."
“In this era of ever more-expensive particle accelerators,
MICE points the way to a new generation of cost-effective muon colliders,” said
Professor Dan Kaplan, Director of the IIT Center for Accelerator and Particle
Physics in Chicago.
Professor Paul Soler from the University of Glasgow and UK
Principal Investigator said: "Ionization cooling is a game-changer for the
future of high-energy muon accelerators, such as a muon collider, and we
are extremely grateful to all the international funding agencies, including
STFC in the UK, for supporting the experiment and to the staff at the ISIS neutron
and muon source for hosting the facility that made this result possible.”
Dr Chris Booth, of the University of Sheffield, who led the
development of the particle-production target on ISIS, said: “The techniques
demonstrated by the MICE experiment will allow new particle accelerators to be
constructed which could explore fundamental physics in areas ranging from
neutrinos to the Higgs boson and the energy frontier that lies beyond the reach
of the LHC.”
Dr. Maurizio Bonesini, who led the effort of the National
Institute for Nuclear Physics (INFN) Italy, to develop the high-performance
particle-identification system of the experiment, said: "It is a great
pleasure to see the successful completion of such an interdisciplinary effort
pointing to a fruitful international collaboration in the field of new accelerators."
Professor Emeritus John Cobb of Oxford University Physics
Department and the John Adams Institute for Accelerator Science, who jointly
led the design and operation of one of the superconducting magnets, said: “Even
though the physics underlying ionization cooling has been understood for many
years, its practical implementation is extremely difficult. MICE successfully
confronted the formidable technical challenges of designing and operating a
system of coupled, high-field superconducting magnets, subject to potentially
enormous forces, which tightly enveloped a delicate vessel containing many
litres of (potentially explosive) liquid hydrogen. The practical experience
gained will be invaluable to the further development of muon accelerators.”
Professor Jingyu Tang of the Institute of High Energy Physics,
Chinese Academy of Sciences who led China’s participation in MICE said, “We are
happy that China contributed to this fascinating project. We all benefit from
the international efforts in the execution of the MICE experiment.”
Professor Yoshitaka Kuno of the University of Osaka, leader
of the Japanese team that designed and built the liquid hydrogen absorber
congratulated the collaboration on its fantastic success in demonstrating muon
ionization cooling and said: "It is a great step towards a future muon
collider. The ionization cooling technique can also be used to make a cold muon
beam to enhance significantly the future development of low-energy muon
physics, including searches for charged lepton flavour violation through the
observation of
.”