Perhaps the most developed area of muon collider research is into proton targets. Past and ongoing studies aim to find a robust target which can withstand the high radiation, temperatures and pressures applied by the powerful proton beam, ensuring a consistently high yield of muons. These studies also explore the intense solenoidal fields needed to focus collision products. Like the targets, the magnets generating these fields must also tolerate this punishing environment.
The MERcury Intensive Target (MERIT) experiment, conducted in 2007, demonstrated a feasible target to produce intense muon beams. Proton beams from CERN’s proton synchrotron were directed at a liquid Mercury target, selected for its high atomic number and resistance to melting, unlike solid alternatives [6]. Despite its success in yielding muons, the liquid mercury target is hazardous to handle and susceptible to damage.
Researchers at the University of Warwick and Rutherford Appleton Laboratory (RAL) are seeking preferable alternatives. The collaboration has designed and tested an innovative system which circulates a medium containing Tungsten Powder. Circulation of this dense material reduces damage, increasing durability of the target. Having conducted an initial beam experiment, they hope to optimise the system in preparation for beams of even higher power [7].
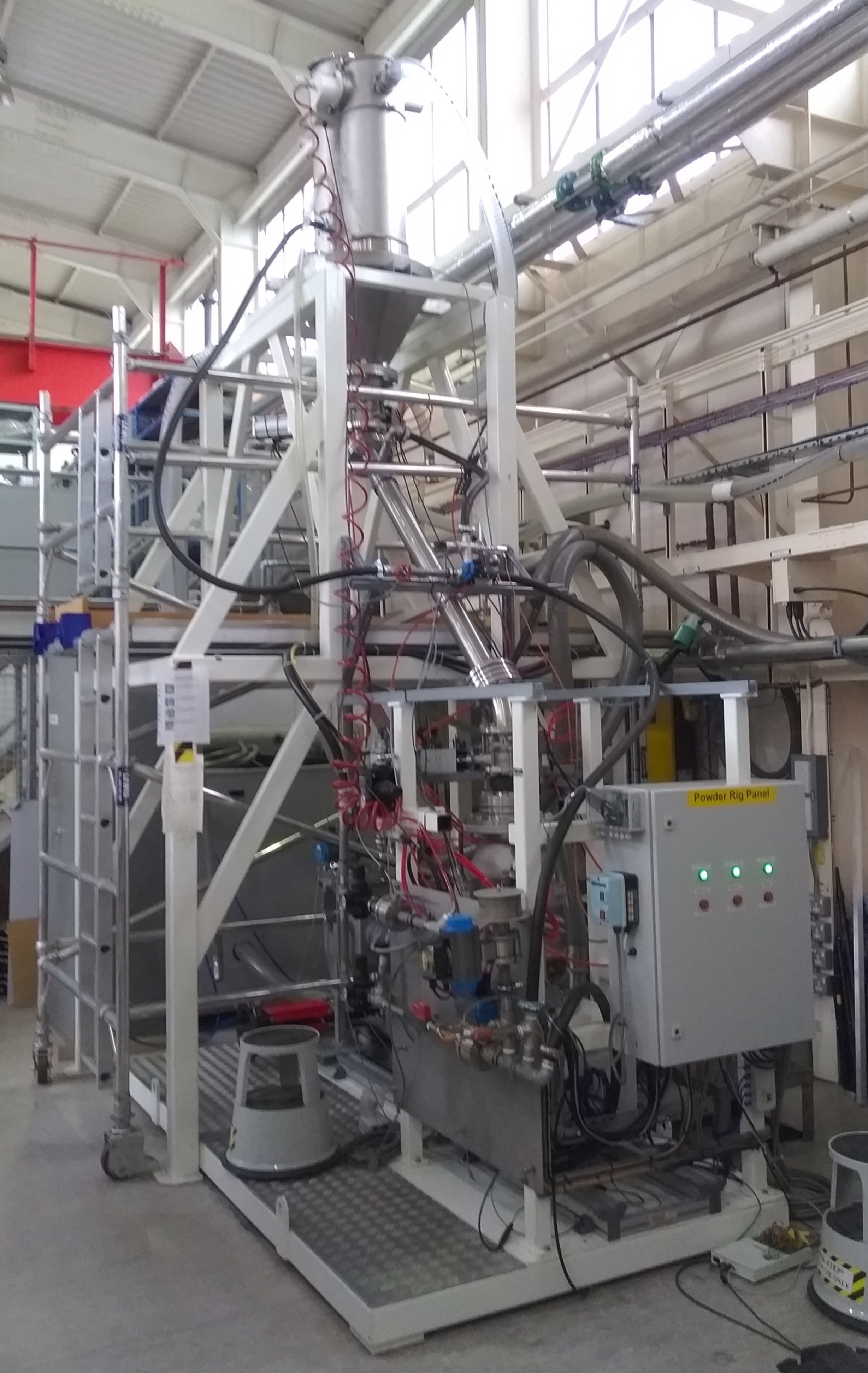
Tungsten powder handling rig at RAL
After the proton beam strikes its target, the resulting muons must be concentrated into a beam and be accelerated for collision. This requires the initially diffuse cloud of muons to be focused into a narrow, pencil-shaped beam. This process of emittance reduction, also known as cooling, is constrained by the muon's short lifetime. Ionisation cooling can provide a rapid and effective solution.
After the production and isolation of muons, the high intensity beam is focused onto a component called an absorber via superconducting solenoids. Prior to their entry, muons occupy a large space due to an average high momentum, perpendicular (transverse) and parallel (longitudinal) to the direction of travel.
The absorber ‘absorbs’ this momentum, with the muons interacting with liquid within the absorber. By liberating electrons from their nuclei, the muons lose kinetic energy. Collision with nuclei has the opposite effect: this is minimised through the selection of less dense materials such as liquid hydrogen or lithium hydride.
After passing through the absorber, momentum in both planes are reduced, shrinking the space they occupy. Whilst this focuses the beam, it lowers the longitudinal momentum and muons short lifetime necessitates rapid acceleration. Restoration of its longitudinal momentum occurs through the alternating electric fields of a Radiofrequency (RF) cavity. This cooling effect accumulates as the process is repeated, with the initially chaotic muon cloud reduced to dense bunches. Eventually, by passing through multiple absorbers and RF cavities, this tight bunch of muons can be efficiently accelerated.
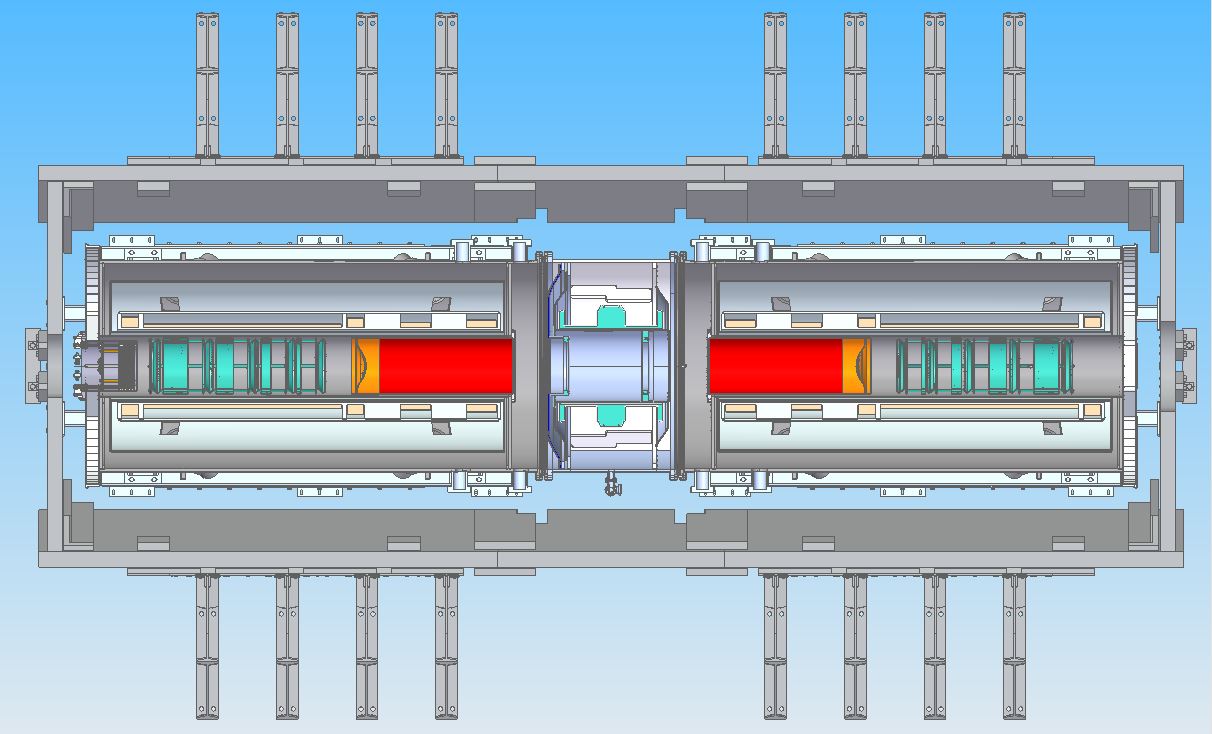
Rendering of single cooling cell and its surrounding steel shielding: Entering the cell, they travel through the tracker (cyan), tracing momentum and measuring momentum. Superconducting solenoids focus (presence indicated by orange and red) muons onto absorber (light blue component in the center).
The Muon Ionisation Cooling Experiment (MICE) is an international research collaboration, using a muon beam at the RAL ISIS Neutron and Muon Source, that intended to demonstrate this effect. A muon beam was directed to an absorber without acceleration, measuring important parameters such as emittance and muon density. MICE was successful, serving as the first demonstration of ionisation cooling. The collaboration observed a reduction in transverse momentum and increase in muon density [8].
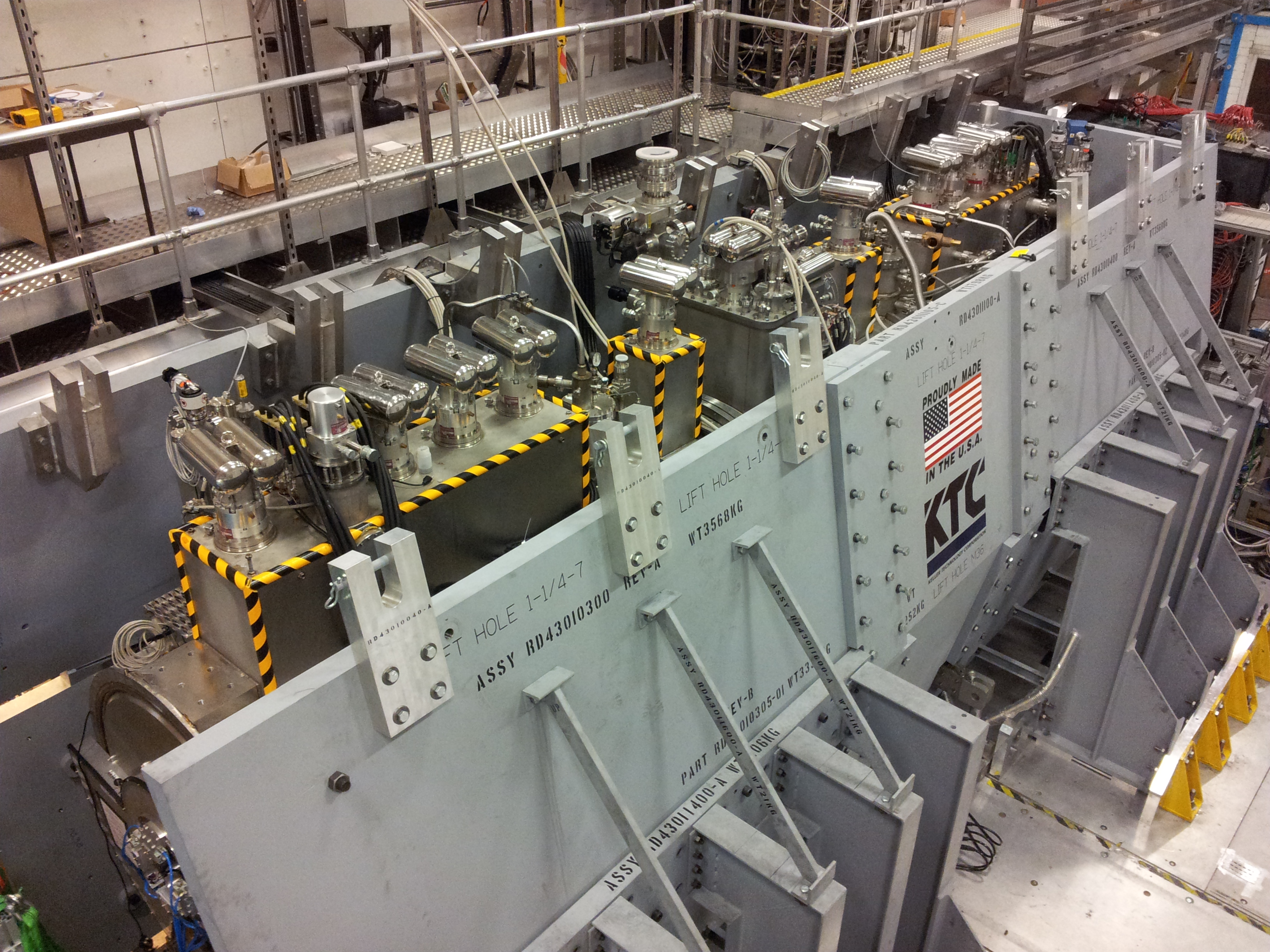
MICE apparatus at the STFC ISIS Neutron and Muon Source. This is where the experiments demonstrating ionisation cooling took place from 2017 to 2019. Metal panels shield computing infrastructure from strong solenoidal fields. Image credit: STFC ISIS
The IMCC aims to build on the achievements of the MICE collaboration by developing a future demonstrator experiment that more closely replicates the conditions required for a muon collider. This next step would involve demonstrating the reduction of both longitudinal and transverse momentum across multiple absorbers. Additionally, it would need to operate with a higher-intensity muon beam and accommodate a wider range of emittance values [9]. A successor to the MICE experiment, capable of cooling in both planes with multiple absorbers, first requires advancements to magnet engineering, RF cavities and cooling technology.
Muons emerge from the cooling apparatus primed for acceleration, tightly bunched and strongly focused. IMCC envisages a low energy muon acceleration via a series of linear accelerators followed by injection of the beam into a synchrotron.
Once injected, they will undergo further acceleration to relativistic velocity before collision. Conventional pulsed synchrotrons are a potential option for high energy acceleration though more efficient and cost-effective alternatives exist [9].
Rapid Cycling synchrotrons and Fixed Field Alternating Gradients (FFAs) are both promising alternatives. FFAs are particularly appealing as they can rapidly accelerate particles from a broad range of initial energies. This feature makes them well suited to applications like muon colliders. The Electron Model for Many Applications experiment (EMMA) demonstrated the feasibility of a subset of FFAs at low energy. Commissioned in 2010 at STFC’s Daresbury laboratory, EMMA accelerated electron beams on a timescale compatible with muon lifetime. Significant adaptation is needed to scale this technology for use within high energy muon beams [10].
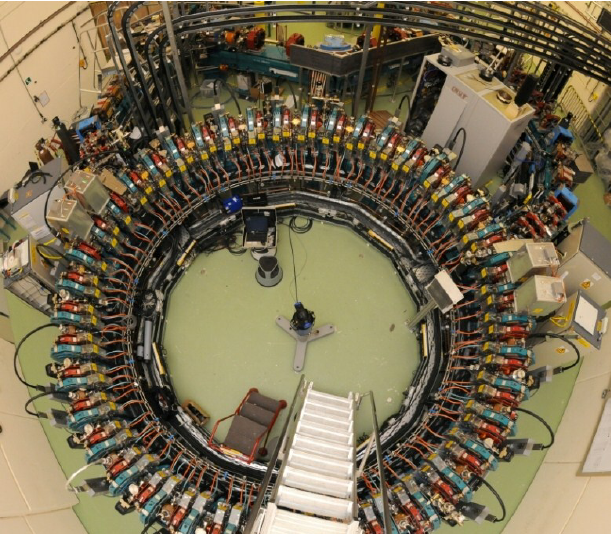
Image credit: EMMA experiment at Daresbury Laboratory. World’s first non-scaling FFA accelerator The EUROnu Project (2013) DOI: Link. Figure 13
Muon decay: Detection issues and damage to components
Entering the collider ring, the two opposing muon beams are set to collide at the TeV energy scale. Despite experiencing time dilation, muon survival wanes, with estimates of 10% loss by this stage. Many muons decay to produce electrons, positrons and neutrinos. These electrons and positrons will deposit their energy into the surrounding collider components, leading to radiation damage. IMCC is exploring technology that would tolerate / protect against the energy deposited [11].
Research on mitigating muon decay products is also relevant to particle detectors. These complex systems must be able to isolate the collision products from the signatures produced from muon decay known as Beam Induced Background (BIB). Detectors must be able to sift through vast swathes of BIB without compromising the desired events [11].
Preliminary designs for the detector have been produced and used to create simulations accurate for muon collision at 1.5 TeV. These simulations shed light onto challenges that BIB poses to muon colliders and offer feasible solutions to BIB mitigation. IMCC will first ensure these encouraging results are replicated at higher energies, up to 10 TeV, before finalising detector design.
Balancing Ambition with Reality: The future of Muon colliders
Several different colliders have been proposed for the future, with a variety of different technology choices and at many different locations. At the time of writing none of these has been approved for construction, and there will be an interesting few years ahead as the decision process across laboratories, review panels and national funding agencies continues.
Muon colliders are an appealing candidate, boasting an impressive precision and high Center of Mass energy. The creation of a bright muon beam has applications for neutrino physics and muon spectroscopy, development towards muon colliders will stand to benefit these synergistic fields. A muon collider would have lower space and power requirements than an electron or proton collider of comparable physics reach, but, as discussed here, requires the development of several novel technologies. Conversely, competing facilities are at more advanced stages of planning, while muon colliders are yet to settle on a single design or select a construction site [12].
The IMCC is working to overcome the many technical barriers to present a convincing case for the next European Strategy for Particle Physics Update in 2026 [12]. If successful, the IMCC proposal outlines continuation of their comprehensive R&D program, followed by integration of these technologies in large scale demonstrator experiments, ready for possible construction to begin in 2045 [12].
[1] Long, K.R., Lucchesi, D., Palmer, M.A. et al. Muon colliders to expand frontiers of particle physics. Nat. Phys. 17, 289–292 (2021). https://doi.org/10.1038/s41567-020-01130-x
[2] IMCC collaboration - Towards a Muon Collider (2023) Link
[3] Roman Pöschl Future colliders – Linear and circular colliders (2018) Proceedings of ALPS2018
[4] Murayama, Hitoshi, Asai, Shoji, Heeger. et al Exploring the Quantum Universe: Pathways to Innovation and Discovery in Particle Physics. (2023) doi:10.2172/2368847. Sections 5.1.4 - 5.1.5 then Section 4.1
[5] Kyberg et al nuSTORM Neutrinos from STORed Muons. Letter of Intent to the Fermilab Physics Advisory Committee (2012) Link
[6] I, Efthymiopoulos, MERIT collaboration, MERIT - The high intensity liquid mercury target experiment at the CERN PS 2008 IEEE Nuclear Science Symposium, Medical Imaging Conference and 16th Room Temperature Semiconductor Detector Workshop pp 3302 – 3305 DOI: 10.1109/NSSMIC.2008.4775051
[7] Chris Densham Potential of a Circulating Fluidised Bed (CFB) as a Muon Collider target Indico Link
[8] MICE collaboration Demonstration of cooling by the Muon Ionization Cooling Experiment (2020) Nature, 578, 53 – 59
[9] Daniel Schulte, Muon collider DOI: Indico. Youtube Link
[10] J. Scott Berg FFAs for Acceleration in a Muon Collider (2024) Indico Presentation: Link
[11] Dr Karol Krizka Building the case for a muon collider (2024) Link
General References
- Daniel Schulte Muon Collider (2024) Link
Great presentation: Establishes potential IMCC strategy and objectives.
- Muon collider Snowmass White Papers (2022) - Link
Used for 3 TeV feasibility study and promising technologies.
- IMCC website (CERN) - Link
Basic overview. Navigate Structure / Resources for more detailed studies
- F Collamati, C Curatolo, D Lucchesi, A Mereghetti, N Mokhov, M Palmer, P Sala Advanced assessment of Beam Induced Background at a Muon Collider (2021) Link
Interesting paper about BIB strategies in detectors in 1.5 TeV simulations.