For over 40 years, dark matter has eluded experiments at dedicated experiments such as LUX-ZEPLIN or within searches conducted at ATLAS and CMS. These experiments are often guided by searches for Weakly Interactive Massive Particles (WIMPs), WIMPs occupy a vast mass range from 100 –10,000 GeV.No clear candidate for dark matter has emerged, prompting physicists to search beyond this mass range. Many models with a mass range on the order of a proton (1 – 10 GeV) are exempt from these searches. Yet there is strong motivation to search within this parameter space, with verification of these models helping to explain our universe's matter / antimatter asymmetry.
The QUEST-DMC collaboration draws upon expertise from condensed matter physicists and particle physicists to produce a superfluid 3He detector, maintained at ultra-low temperatures (> 300µK).
The experiment is hosted at Royal Holloway, University of London, in collaboration with researchers from Lancaster, Oxford, Liverpool, and Sussex universities, as well as STFC and the National Physical Laboratory. The experiment is funded by STFC and EPSRC as part of Quantum Technologies for Fundamental Physics (QTFP).
QUEST-DMC Experimental Mechanism
QUEST-DMC will utilise novel quantum technology to impose world leading constraints on sub-GeV dark matter candidates, probing spin-dependent interactions with 3He. The superfluid 3He target is contained within an 1cm3 bolometer, instrumented with vibrating nanowire resonators. Since the bolometer is placed within a magnetic field, as an alternating current flows through the nanowire, the nanowire will vibrate at its resonance frequency. When an incoming particle, such as a dark matter particle, scatters from a 3He nucleus in the target, this will generate deposit in the superfluid target through heat (from quasiparticle production), and excitation/ionisation (more on the latter below). Since the nanowire is sensitive to the quasiparticle density, its increase prompts a change to this frequency. Alterations in frequency are marked by change in voltage of the nanowire over time. So far, QUEST-DMC has operated two experiments using this principle, and the collaboration is now in the process of cooling the third experiment, to further develop and validate this experimental technique.
Two QUEST-DMC bolometers - Two bolometers are shown next to a ruler. Looking along its width you are able to see they measure 10mm, comparable to the size of a 1p coin.
Dark matter experiments are typically hosted underground, where layers of rock and earth serve as a natural shield from cosmic rays, the primary source of background interference. QUEST-DMC operates at ground level, where cosmic rays constitute the majority of background noise. To attain the exacting measurements for these extremely low energy thresholds, this source of noise must be mitigated.
Established techniques in dark matter and neutrino experiments help mitigate interference from cosmic ray muons by surrounding the detection medium with scintillating plates. When high energy muons pass through, they produce scintillation photons which can be detected by photon detectors. These unwanted signals are then filtered based on the timing and angle. QUEST-DMC will use this strategy to reject cosmic ray backgrounds through novel photon detection technology for operation at ultra-low temperatures.
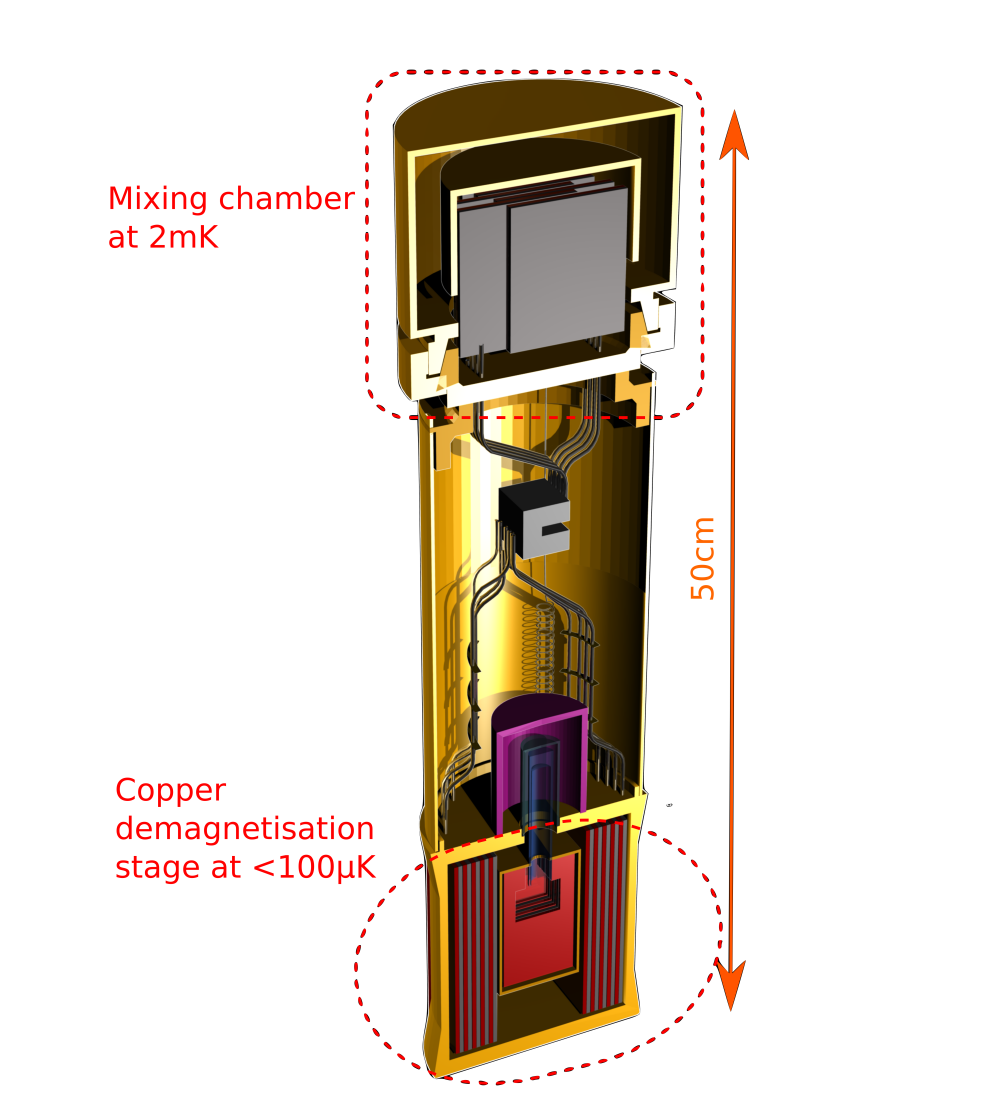
QUEST-DMC experimental apparatus - At the top, a mixing chamber contains a pool of 4He - 3He at 2mK. The lines from this chamber to the bolometer, the temperature lowers to a fraction above absolute zero. The nascent 3He is stored within the area highlighted by the dotted red circle.
Above this red area is the transparent 3He bolometer, surrounded by the Silicon Photomultipliers (represented as the surrounding purple shell).
Image from QUEST-DMC collaboration publication
PPD Involvement
Silicon Photomultiplier R&D
Scintillating plates need to be positioned close to the photon detectors to preserve the spatial and timing resolution, requiring their operation at ultra-low temperatures—though on the order of millikelvins, rather than microkelvins. The Particle Physics Department is currently conducting R&D on photon detectors designed to function under these extreme conditions. This includes evaluating various types of photon detectors, such as Silicon Photomultipliers, and exploring different scintillator and readout configurations.
3He Scintillation and Particle Identification
Elastic scattering interactions with 3He atoms deposit energy through heat (quasiparticle production), and through the excitation and ionisation of atoms, forming excimers. Excimers are short-lived excited ion dimers that decay into one of two ground states, termed singlet or triplet, via the emission of UV photons. The ratio of heat-to-scintillation produced is determined by the type of interaction that has occurred, an interaction with the nucleus causing a “nuclear recoil”, or an interaction with a bound atomic electron causing an “electron recoil”.
In the case of QUEST-DMC, dark matter signals are expected to induce nuclear recoils, whilst background particles from natural radioactivity will induce electron recoils. If QUEST-DMC were sensitive to the UV photons as well as the quasiparticles, the different fractions of energy in photons and quasiparticles for electron and nuclear recoil events could potentially be used to discriminate between these two interaction types. In addition, below the ionisation energy of 19.7 eV, all the energy will go into the quasiparticle channel and for a dark matter search below this energy, the photons can be used as a veto to reject higher energy background events.
Signal discrimination methods
The decay lifetime of an excimer depends on whether the transition occurs to the singlet or triplet state. In other noble liquid gases, notably liquid argon, the difference in the lifetimes of singlet and triplet states can be up to as much as three orders of magnitude. Since the ratio of singlet-to-triplet states is also different for nuclear recoils versus electron recoils, they have very different time distributions. This has yielded a very powerful background discrimination tool in liquid argon-based experiments known as “Pulse Shape Discrimination” (PSD), where the timing profiles are used to easily distinguish nuclear (signal-like) recoils and electron (background-like) recoils.
Following the development of a reliable muon veto, PPD will look to design and develop an upgraded photon detection system capable of detecting scintillation originating from the 3He target itself, in the hope that particle identification based on energy partition, or PSD, can be used to substantially enhance QUEST-DMC's sensitivity to dark matter.