In commemoration of CERN’s 70th anniversary, this article will reflect upon the UK wide contribution to CERN from its founding to ongoing HL-LHC upgrades. This shining example of scientific collaboration has led a fruitful history of landmark discoveries and spin off applications. This retrospective will detail some highlights of CERN’s history and what role the UK has fulfilled.
70 Years of collaboration
Origin of our association
The UK was a founding member state for CERN and has since been heavily involved in the physics conducted at CERN. It may be surprising to hear then, that the initial idea to join a European laboratory was met by some skepticism within Britain’s nuclear physics community [1],[2]. Whilst the US was at the forefront of nuclear research, Britain's capability was considered advanced at the time, with simultaneous operation and planned construction of several high energy accelerators. Innovative experimental programmes being conducted at laboratories including the Atomic Energy Research Establishment (Harwell, near Oxford) meant the UK initially lacked enthusiasm to join as a full member state.
Prominent UK physicists were active supporters of the proposal, and their advocacy fostered British enthusiasm for the organisation [1]. Thanks to their efforts, Britain was among 10 other countries to sign the first resolution concerning the establishment of a European Council for Nuclear Research in 1951. After 3 years of planning and coordination, this provisional organisation was signed into being on the 29th of September 1954.
This date marked a new type of international scientific collaboration, and the world’s first fully international laboratory, dedicated to uncovering the fundamental laws of physics. Today, the collaboration receives input from a vast community of researchers from 70 different countries. Member states pay an annual membership fee, proportional to their national income, for the construction and operation of CERN facilities. In return for their investment, member states get a say in CERN’s decision, participation in science conducted, employment and training opportunities as well as the potential for commercial contracts. [3]
UK nationals make up a substantial proportion of CERN staff. Additionally, researchers from over 30 universities and national laboratories facilitate the UK’s invaluable input, with the full list found here. Over the years, figures from the UK have played a key role in the development of major CERN projects.
The world’s first strong focusing synchrotron
In 1953, the UK sent a team including accelerator physicists John Adams and Mervyn Hine from Harwell to Geneva. They were selected based on their extensive technical experience with the Harwell synchrocyclotron, tasked with helping to solve the formidable problems associated with the proposed proton synchrotron (PS) project. The decision to incorporate alternate gradient focusing offered a tantalizing leap forward in particle energy with smaller, less expensive magnets, but it would prove very difficult to implement. Optimism quickly turned to frustration as the beam proved to be very sensitive to small changes in the magnetic field of the accelerator. Adams and Hine became known as ‘the terrible twins’ for their ‘Jeremiah like prognostications about inhomogeneities, that became a depressing feature of PS meetings’. John quickly rose to become director of the PS project. [2]
Under Adams’ calm assurance and engineering expertise these complicated technical issues were addressed, and the proton synchrotron project was completed as scheduled in 1959, below budget. It is still used today, not least as an early link in the chain of accelerators leading to the Large Hadron Collider.
John Adams, leader of the proton synchrotron construction team, holding an empty bottle of vodka. The bottle had instructions that it was to be drunk immediately following the beams generated by the proton synchrotron surpassing the world record held by the USSR’s Dubna facility. The proton synchrotron was briefly the world's most powerful particle accelerator.
Neutral currents discovery
During John Adam’s and delivery of large bubble chambers among several CERN member states. Bubble chambers were used extensively in the UK and other countries, with several installed at NIMROD, the 7 GeV particle accelerator at the Rutherford High Energy Laboratory (RHEL, now the Rutherford Appleton Laboratory (RAL).
Commissioned in 1964, NIMROD was a major facility for UK particle physics, conducting studies in parallel with CERN. Research institutions would propose and run experiments, receive the results in film reels and transport them back to the UK to have them processed. Dedicated teams would manually analyse the interactions on the photographic film. Their hard work, sometimes even overnight, was able to characterize a number of important interactions.
Bubble chamber and spark chamber analysis teams were gradually phased out in favour of computing-based automation. UK computing centres, including those at Harwell, were among the fastest and most powerful computing systems at the time. Their vast processing capabilities were used for data analysis of CERN’s bubble chamber experiments.
The 1960s also saw the first foray into high field superconducting technology with great strides being attributed to UK laboratories. Substantial progression was made with record breaking DC superconductor prototypes, innovations within cryogenic technology as well as establishing the basis for superconducting magnets worldwide, Rutherford cabling [4], [5].
The search for the W and Z bosons
A group from University College London analyzing neutrino interactions for the CERN bubble chamber, Gargamelle, were part of the collaboration finding evidence of neutral current interactions in 1973 [7]. This was a major prediction of the electroweak theory, a unification of electromagnetism and weak nuclear interactions. The theorists who proposed electroweak theory (Sheldon Glashow, Abdus Salam and Steven Weinberg) later won a Nobel Prize for this work following its verification at CERN. [6]
In the 1970s there were high hopes for CERN’s future, with the completion of several major projects. This included the world’s first hadron collider, the Intersecting Storage Rings, and later the Super Proton Synchrotron (SPS).
The operation of these new facilities would coincide with the closure of NIMROD in 1978. The focus of UK particle physics had already largely shifted from domestic experiments to research at CERN and other large laboratories. RAL, the Daresbury Laboratory in Cheshire and UK universities worked together to use their extensive computing and technological assets to contribute to CERN’s experiments. [8]
After directing the UK nuclear fusion programme at the Culham Laboratory, John Adams returned to CERN in 1971 as Director-General. His competent direction had seen the PS built and now he oversaw the operation of its successor, the SPS. Initially, beams of protons were directed at fixed targets, but this was later upgraded, to make the SPS collide proton – antiproton beams. Collisions would occur in experiments including UA1 and UA2. [9]
Carlo eader of the UA1 team, offered a modest description of the UA1 detector. He described it as 'a series of boxes, each one doing what the previous one couldn't do': a simple way to explain an immense 2000 tonne detector. A significant portion of this ‘series of boxes’ was assigned to the UK. [10]
The UK was responsible for the manufacture of the trigger system. A sophisticated computer would select important events based on preprogrammed criteria. Thousands of collisions occurring each second would generate enough raw data, from all detector compartments, to fill several book volumes. Without triggering and subsequent data handling, this would have overwhelmed the digital storage of the time.
In addition to the trigger, several UK institutions coordinated the design and manufacture of the huge UA1 hadronic calorimeters, precisely measuring particles experiencing the strong nuclear force.
High precision was also needed to hunt for the W and Z bosons. In particular, the W boson would often decay to an electron and a neutrino: the elusive neutrino was not seen directly but inferred from momentum calculations.
Through the fantastic feat of data handling and successful functioning of the trigger processor, the thousands of millions of events recorded had been reduced to just 4 and 6 for the UA1 and UA2 respectively. These events provided sufficient evidence to officially announce the W boson, in 1983. With the momentum from this discovery, they continued their analysis, with improved methods and techniques. Only 2 months following the W boson announcement, luminosity had soared to 100 times that of its original run and a Z boson candidate was evident [10].
This discovery heralded a new era for particle physics. The W and Z bosons finally confirmed electroweak theory, suggesting that something must be providing force carriers with mass – perhaps via the Higgs mechanism.
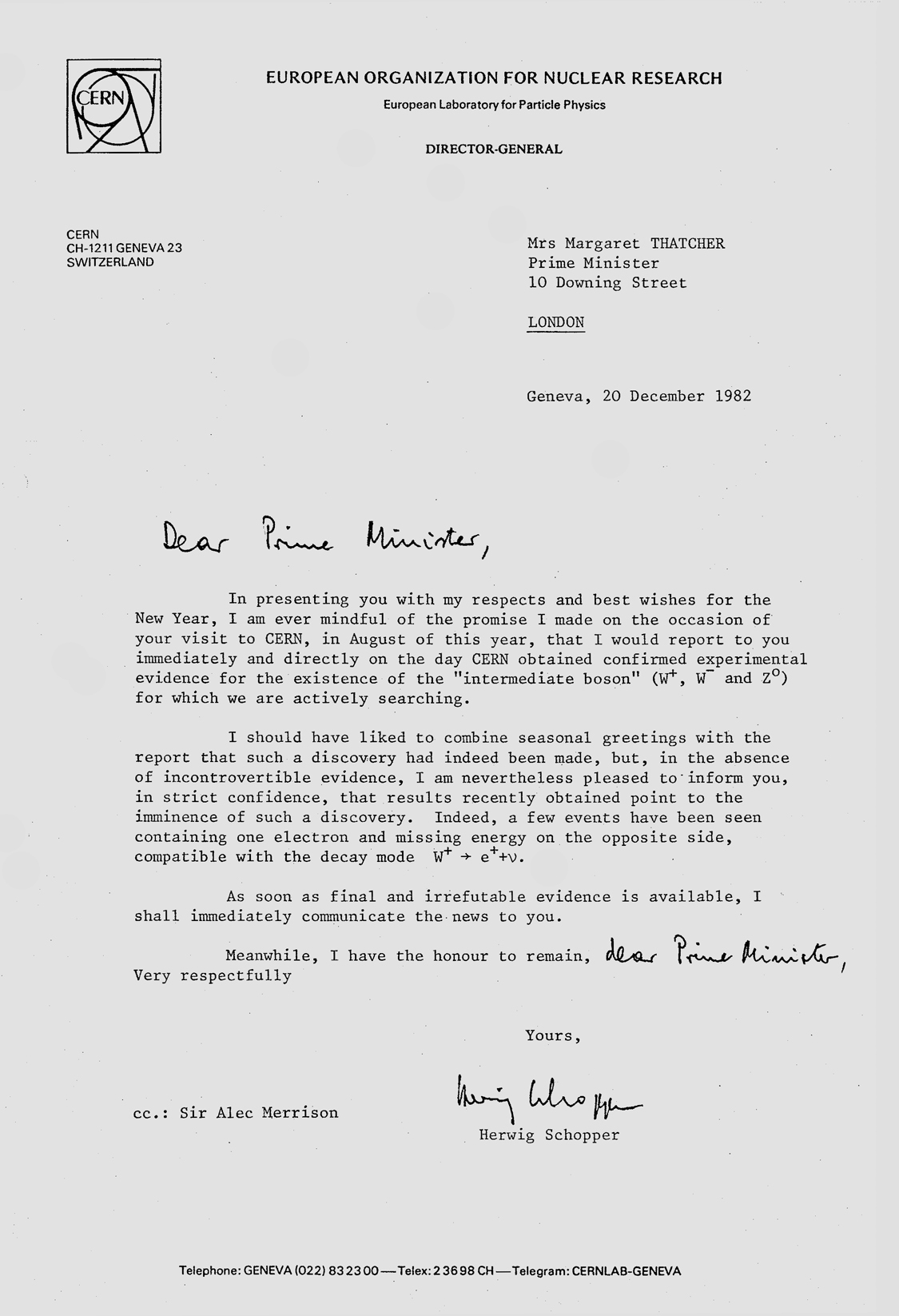
This epic discovery enthused the public, in much the same way as the Higgs Boson discovery in 2012 did. Margaret Thatcher, UK PM at the time, requested that she would be informed personally of its discovery by the Director General of CERN. Telegram courtesy of CERN Document Server
The world's largest superconducting magnet
Shortly following this monumental achievement, tunneling machines would begin boring the 27km tunnel for the Large Electron – Positron (LEP) collider. Set to expand on the SPS’s findings, over 2 runs spanning 1989 to 2000. The LEP would provide precise measurements for W and Z bosons and constrain deviations from the Standard Model of particle physics. for various
A highlight of the UK contribution to LEP was the construction of the DELPHI superconducting coils. DELPHI would need to generate an immense magnetic field allowing for precise measurements of Z decay to take place. RAL, with its long-standing expertise in the field of superconductivity, was responsible for its design and manufacture [11]. Elwyn Baynham, the division head of the Applied sciences division at RAL, recalled the difficulty in its transport to CERN.
.jpg)
The DELPHI solenoid leaving RAL. From here, DELPHI was transported at a ‘snail's pace’ to Southampton.
‘The superconducting solenoid was so massive it wasn't suitable for motorways, so it had to take a very ‘scenic’ route through villages from RAL to Southhampton. The journey was further complicated by the massive storm of October 1987 so a journey that’d ordinarily take 2 – 3 hours by car instead took 2 – 3 weeks. They had a convoy to help move power lines overhead so that they could pass along the small roads.
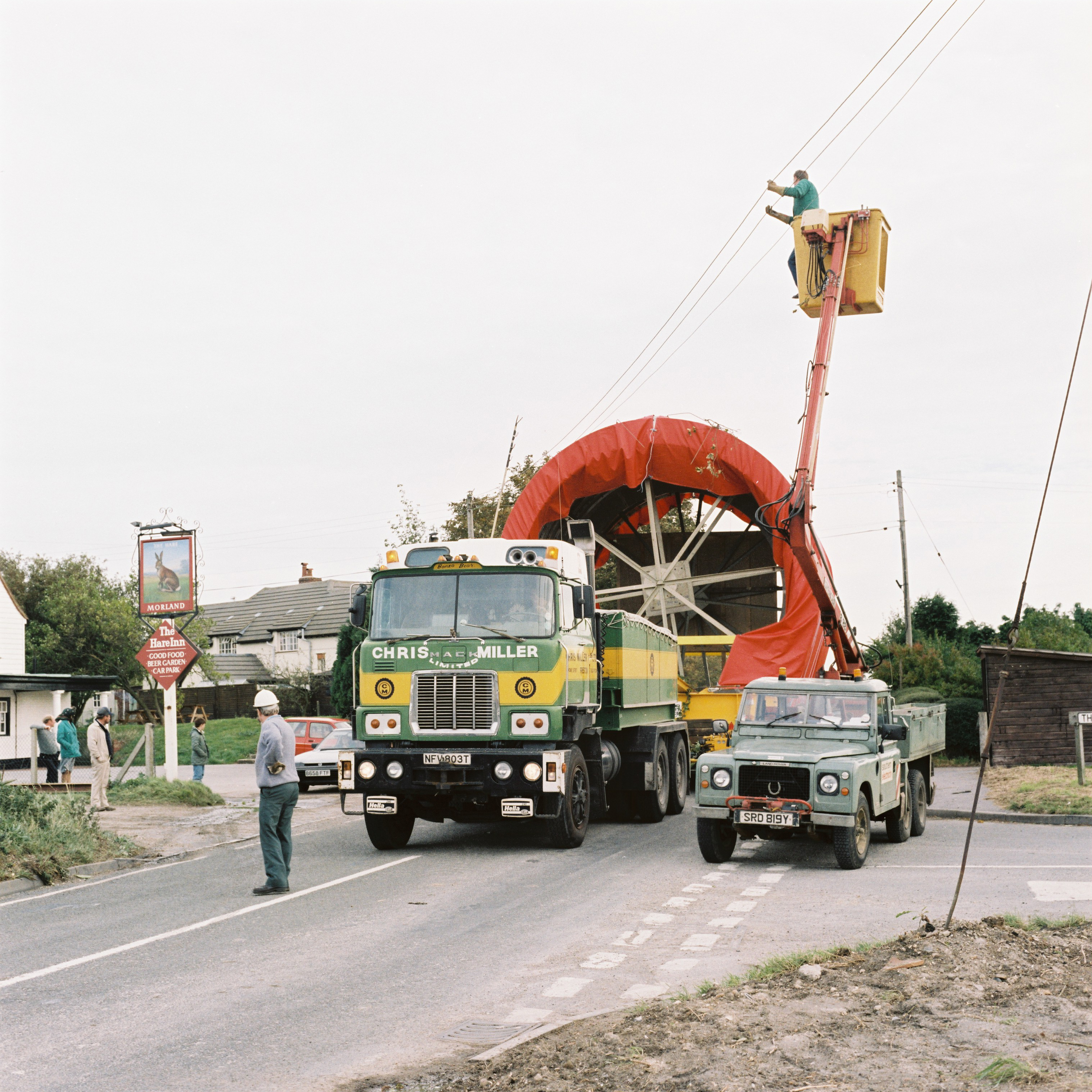
The DELPHI magnet shortly after leaving RAL. A car of the following convoy can be seen moving overhead wires to allow the gigantic magnet to pass through. At the time, DELPHI was the world’s largest superconducting solenoid.
Baynham recalls a conversation with a colleague who claimed people would be interested to see the DELPHI superconducting solenoid passing through their towns/ villages. However, when the truck carrying DELPHI had disconnected the power lines of a pub at lunchtime the patrons were evidently not so pleased.
Eventually after travelling overseas, across rivers and over mountains DELPHI would complete its 1600km journey and begin operation in 1989. Its operation exceeded the intended design parameters with data taking concluding in 2000.
Aside from construction, the UK was instrumental in the exploitation of these detectors. Within OPAL for instance, groups from the UK helped to establish lower bounds on the top quark mass [12].
Surmounting the challenges of LHC
In 1994, the go ahead for the LHC was received. Preliminary planning and designing had already taken place, with work well underway to replace the LEP within its 27km tunnel. Many aspects of the design, planning and manufacture of this gigantic project came from key UK figures such as Chris Llewellyn Smith, the Director-General of CERN from 1994 – 1998, and Lyn Evans, project manager for the design and construction of the LHC [13].
During the planning for the LHC in the 1990s, it was realized that the current infrastructure was not capable of analysis for the immense data generated. Processing power and data storage from large centralised computers at CERN and national centres were able to contend with the volume of data produced by the LEP, but the LHC was projected to require 100s of times more processing power and data storage for a year of processing [14].
The LHC was designed to collide particles of up to 14 TeV millions of times a second across 4 different experiments. These collisions would allow LHC experiments to find or rule out new particles and better understand the Standard Model. To attain the highest experimental sensitivity possible for this complex data, an overhaul of the current centralised computing systems at CERN and national centres was needed.
Computers and file storage around the world were connected together by networks, allowing researchers to tap seamlessly into far more resources than available at their local laboratories. This system is known as the worldwide LHC computing grid (WLCG), with the UK GridPP collaboration participating since its foundation.
Chris Brew, a grid computing expert at RAL, says ‘STFC continues to play a major role in the maintenance, development and management of the WLCG. It allows us to process and analyse the vast amounts of LHC data. This international network also fosters trust among its users.’
Present day involvement
CERN's flagship facility: The LHC
Finally, after 10 years of construction one of the most advanced scientific instruments in the world was complete: a titanic feat only possible through open collaboration among member and observer states. Fruit of their labours could finally be harvested, with frontier science being conducted across four major experiments (ATLAS, CMS, LHCb and ALICE).
The UK is a member of these experiments and was responsible for the design and manufacture of key detector components. Across all the LHC experiments, the UK specialises in the production of silicon detectors for following charged particles. Dedicated manufacturing facilities, such as RAL’s clean room, fabricate these components within highly controlled environments to limit contamination of these sensitive instruments. Our expertise has been drawn upon from LHC construction through to the ongoing HL-LHC upgrades.
![]()
Examples of silicon detector components manufactured within a clean room. Pictures display prototypes for the silicon strip module (left) and pixel ring(right)
Another area of expertise is within software / hardware triggering, an important aspect of data processing. The UK is a part of the collaboration to build the L1Calo trigger for ATLAS as well as helping to deliver the level 1 trigger at CMS. Triggering involves the incorporation of data from detector components, data is processed to determine whether a collision is significant. Refined event selection amid greater background in the HL-LHC will require several upgrades. [15]
In CMS for instance, development of machine learning neural networks is underway for implementation into the Level 1 trigger. In addition, CMS will integrate and process data from more detector systems. These upgrades will respectively provide greater efficiency despite pile up conditions as well as increasing the accuracy and adaptability of vertex reconstruction.
CMS level 1 trigger system
Of course, data analysis is also a central aspect of the UK’s role, with institutions in the UK helping with the analysis that uncovered the Higgs boson at ATLAS and CMS in 2012, as well as discovery of the pentaquark at LHCb in 2015. The universities of Birmingham and Liverpool are involved in data analysis for ALICE. The universities of Birmingham and Liverpool are involved in data analysis for ALICE Each experiment has and continues to have extensive input from the UK.In the interest of brevity, this will not be a review of all contributions. To find more consult the embedded links and / or references below.
ATLAS and CMS
ATLAS and CMS are large general-purpose detectors investigating various fields of particle physics. Both these versatile machines have similar goals; conducting measurements of the Standard Model such as the top quark and W/Z bosons, and the Higgs boson. They also search for new particles and physics beyond the Standard Model including dark matter.
The UK has played a major role in the construction and maintenance of both detectors. A review of RAL involvement can be found here: ATLAS , CMS.
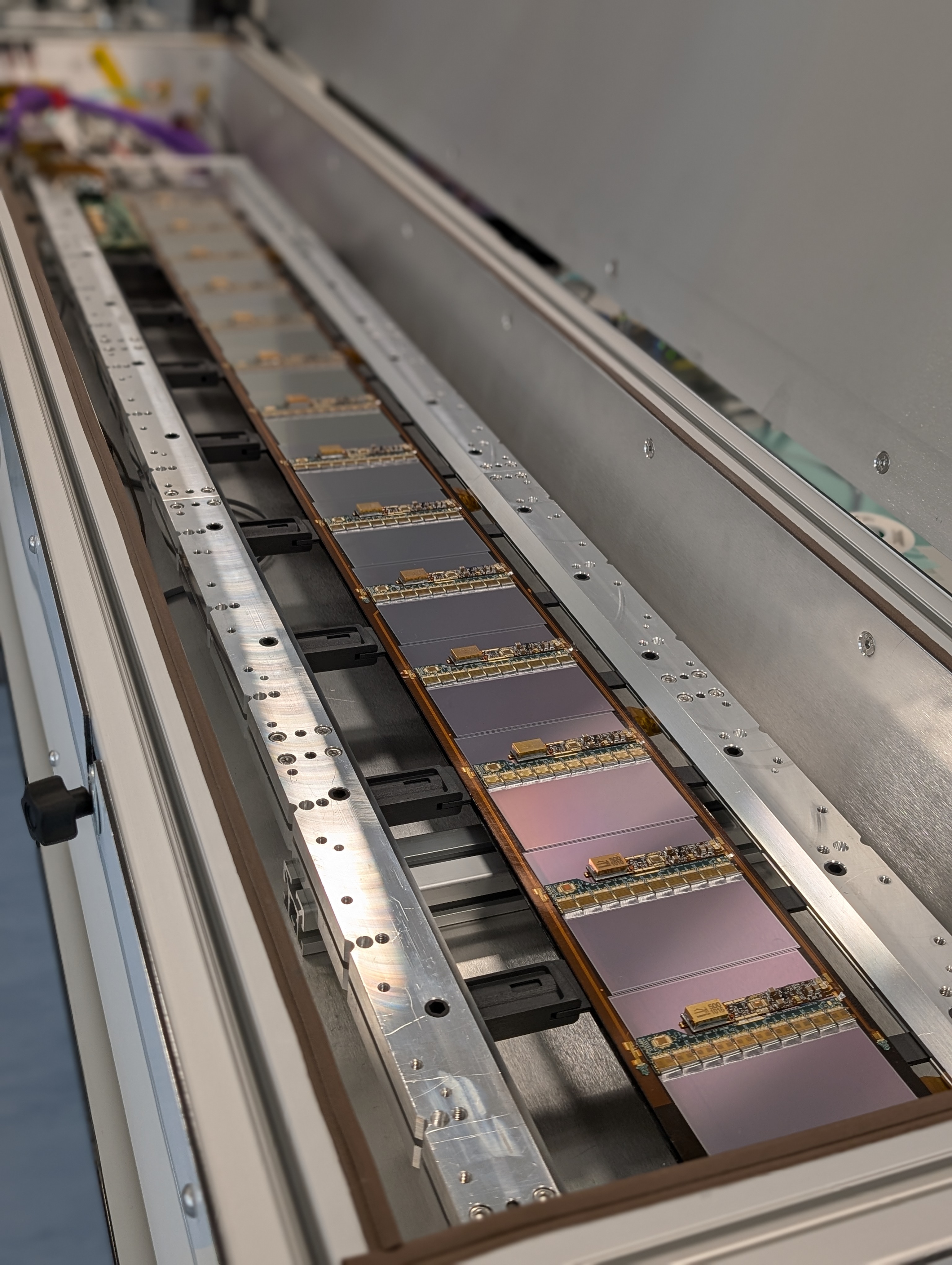
Layers of silicon microstrips are collectively organized into staves. Many staves for ATLAS are manufactured within the UK. For the HL-LHC, the UK is set to deliver 50% of pixel staves for ATLAS.
CMS-UK, along with everything mentioned above, helped to provide the inner layer of the calorimeter, the electromagnetic calorimeter (ECAL). UK RAL manufactured two endcaps for the ECAL and continues to support ECAL operation at CERN.
LHCb is a smaller, more specialised detector aiming to capture as many beauty and charm quark interactions as possible. LHCb aims to explore lepton flavour and number violation. A greater understanding of these concepts may lead to the unravelling of the mystery of matter - antimatter asymmetry: why is there more matter than antimatter in the universe?
The UK has been instrumental in the design of sub - detectors for LHCb including the Ring Imaging Cerenkov Detectors, RICH1 and RICH2. From 2010 - 2019, RICH detectors made use of silicon photon detectors, similar to those used within ITk, which were produced and tested in the UK. During upgrades of LHCb in 2019-2021, the UK played a fundamental role in the replacement of RICH1, readout electronics and the installation tooling required for the replacement of the silicon photon detectors with a different detector type: Multi-Anode Photomultiplier Tubes.
![]()
Installation of RICH2 in 2005.
LHCb-UK aims to repeat this contribution for LS4 for use within the HL-LHC [15].
ALICE
ALICE is an experiment dedicated to replicating conditions of the very early universe. Usually, quarks are bound to each other via gluons, the mediators of the strong force, making up protons, neutrons and other particles. The intense conditions induced by lead ion collision create a novel state of matter known as quark-gluon plasma (QGP), where the quarks and gluons are less coupled. Investigation of this state provides a greater understanding as to the nature of the strong nuclear force.[16]
Silicon pixel detectors were replaced with Monolithic Active Pixel Sensors (MAPS) in 2021, offering greater precision in the detection of heavier quarks (charm, bottom, top) and exotic quark states [17].
The High-Luminosity LHC upgrade will generate more instances of rare phenomena sought out by the ALICE experiment. UK institutions have constructed and are currently developing the trigger software necessary to contend with the higher luminosity [18].
What does the future hold?
High Luminosity phase of the LHC
The high luminosity phase of the LHC is on the horizon, with the testing and manufacture of various detector components and accelerator technologies currently ongoing. Whilst luminosity has been increasing steadily throughout LHC’s lifetime, implementation of these new technologies will see a leap in luminosity by a projected 5x that of LHC initial design luminosity [17].
Greater luminosity will allow for the use of higher quality data, enabling greater precision of measurements. An intriguing aspect of this potential is outlined by Dr Monika Wielers, RAL ATLAS Physics group leader ‘HL-LHC will improve our knowledge of the Higgs by more precise measurements and observe rare Higgs decays. The rate of Higgs production will vastly exceed that from the LHC and so it would be a realistic outcome to observe a triple Higgs coupling or possibly observe something even more exciting, observing any deviation from expectations. Analysis of these events can help us to determine the Higgs field potential shape and provide greater understanding to the electroweak phase transition’. The greater collider performance may indeed reveal new phenomena, beyond our current understanding. Planned searches directed towards new physics include additional Higgs bosons, interactions consistent with SUSY models and searches for dark matter particles [16].
What might the next 70 years bring?
On the 17th of March 1954, within the unassuming fields north of the village of Meryin, construction had begun, plans for a united European research laboratory had finally been put into action. Over 70 years, an international hub of particle physics would emerge from the once agricultural landscape. Each successive facility has been either a novel design or increased in scale, culminating in the vast network of experiments today.
A comprehensive retrospective of the history of CERN cannot be written without consideration of the UK’s strong participation. While we have highlighted several remarkable individuals and experiments, for the sake of clarity, this article is by no means exhaustive, with thousands of academics, students and industrial partners working with CERN over the last 70 years and into the future.
Technology developed in the pursuit of particle physics has been applied elsewhere to impact society globally, CERN serves as a catalyst for advancement of technology within a variety of disciplines. Notable examples include the world wide web, grid computing and spectral X ray imaging.
Proposals around a novel facility, the Future Circular Collider (FCC) are being discussed though as of writing no commitment has been made. Hopefully, we can look back at our eventful past, whether that be discovery of W/Z bosons or the Higgs boson, to endeavor into the unknown and continue to answer the many questions that remain of our universe together.
[15] Interviews conducted at RAL within STFC PPD