Interferometers - sosphisticated quantum sensors - are placed on top of each other and connected via a vacuum system known as a baseline. The experiment exploits the wave characters of atoms, to measure the combined sum of these waves from atomic populations within different quantum states. This superposition is measured by the interferometer, analysis of results may yield evidence of Ultralight Dark Matter and gravitational waves.
The experimental site for a 10m baseline AION detector is planned for the Beecroft building at Oxford University. A diverse team of condensed matter and fundamental physicists from Imperial College London, Oxford, Cambridge,Liverpool, Birmingham, Kings College London Universities as well as Rutherford Appleton Laboratory, are collaborating on this project.
Much like QUEST-DMC, the project is funded through the Quantum Technologies for Fundamental Physics scheme (QTFP). This fund is managed by STFC, on behalf of UKRI. Funding rounds were allocated to the collaboration from 2020 - 2025and they have since applied for further funding from the successor QTFP-2 programme.
If funding acquisition is successful, this experiment will likely begin data taking prior to 2030.
Dark matter is one of the prevailing mysteries within particle physics. Despite making up approximately 25% of all matter within the universe, it has eluded detection since cosmological evidence first appeared in the 1930s. During this time, cosmological observations have been able to measure its gravitational interactions though we are no closer to identifying a viable candidate of a known mass.
Many dark matter searches are motivated by a class of particles that interact with ordinary matter via the weak interaction known as Weakly Interacting Massive Particles (WIMPs). WIMPs have been and continue to be, the subject of many direct detection experiments. Dark matter experiments including LUX-ZEPLIN, alongside searches conducted in proton - proton colliders such as the LHC, have not yet provided evidence for a WIMP particle in the GeV to multi TeV mass range. This has lead physicists to explore alternative viable models. Proposed dark matter models span an extraordinary mass range from ultralight candidates, classed as 10-22 eV to 1 eV, up to the Planck scale, with masses exceeding 1018 eV. The AION experiment will be sensitive to this lower end of the spectrum, with the AION collaboration projecting greater sensitivity to many ultralight models, with their search extending beyond parameter space explored by previous experiments.
Additionally, AION is sensitive to gravitational waves. Einstein posited the concept of gravitational waves in his theory of relativity in 1916, stating that accelerating massive bodies would radiate these waves at the speed of light. He described gravitational waves as a ‘ripple in spacetime’, distorting the fabric of the universe as they pass. This distortion, known as gravitational strain, compresses and strengthens the space it traverses, with this rate of change determined by its frequency.
Collosal cosmological events such as the merger of two primordial black holes in 2015 (an event named GW150914) are a source of these waves. The Laser Interferometer Gravitational-Wave Observatory (LIGO) was capable of detecting the minute strain generated by waves produced during this event. Not only will AION explore gravitational wave parameter space complementary to LIGO but will probe into novel parameter space. It is sensitive to strain from gravitational waves that oscillate with a frequency of a few Milli Hz to several Hz. This observation not only serves as a test for Einstein’s theory of relativity but also provides valuable insight into the early evolution of our universe.
Aerial view of the Handford Site at The Laser Interferometer Gravitational-Wave Observatory (LIGO) - This detector was capable of detecting the minute strain generated by gravitational waves produced from the merger of two primordial black holes in 2015 during an event termed GW150914. Image credit - Caltech / MIT / LIGO Laboratory
To understand how this can help address these fundamental questions in physics, it is essential to first provide context about its mechanism.
Experimental Mechanism
The experimental apparatus is as follows: two interferometers are placed apart from one another and are connected via a 10m vacuum tube. Strontium atoms are cooled to a temperature slightly above absolute zero and sent into this vacuum tube. A set of magnetic coils provide a magnetic guide field along the path of the cold atoms, with two layers of magnetic shielding surrounding the vacuum tube.
The atoms are then launched vertically by several metres and begin a free fall descent. A laser pulse strikes them during their descent, this laser pulse induces a superposition of two energy states – grounded and excited. A second laser pulse then recombines these split states. The superposition of these split states generates a characteristic interference pattern, which is ultimately measured by a final laser pulse. Both ultralight dark matter and gravitational waves can interact with the quantum states, causing deviations to the interference pattern.
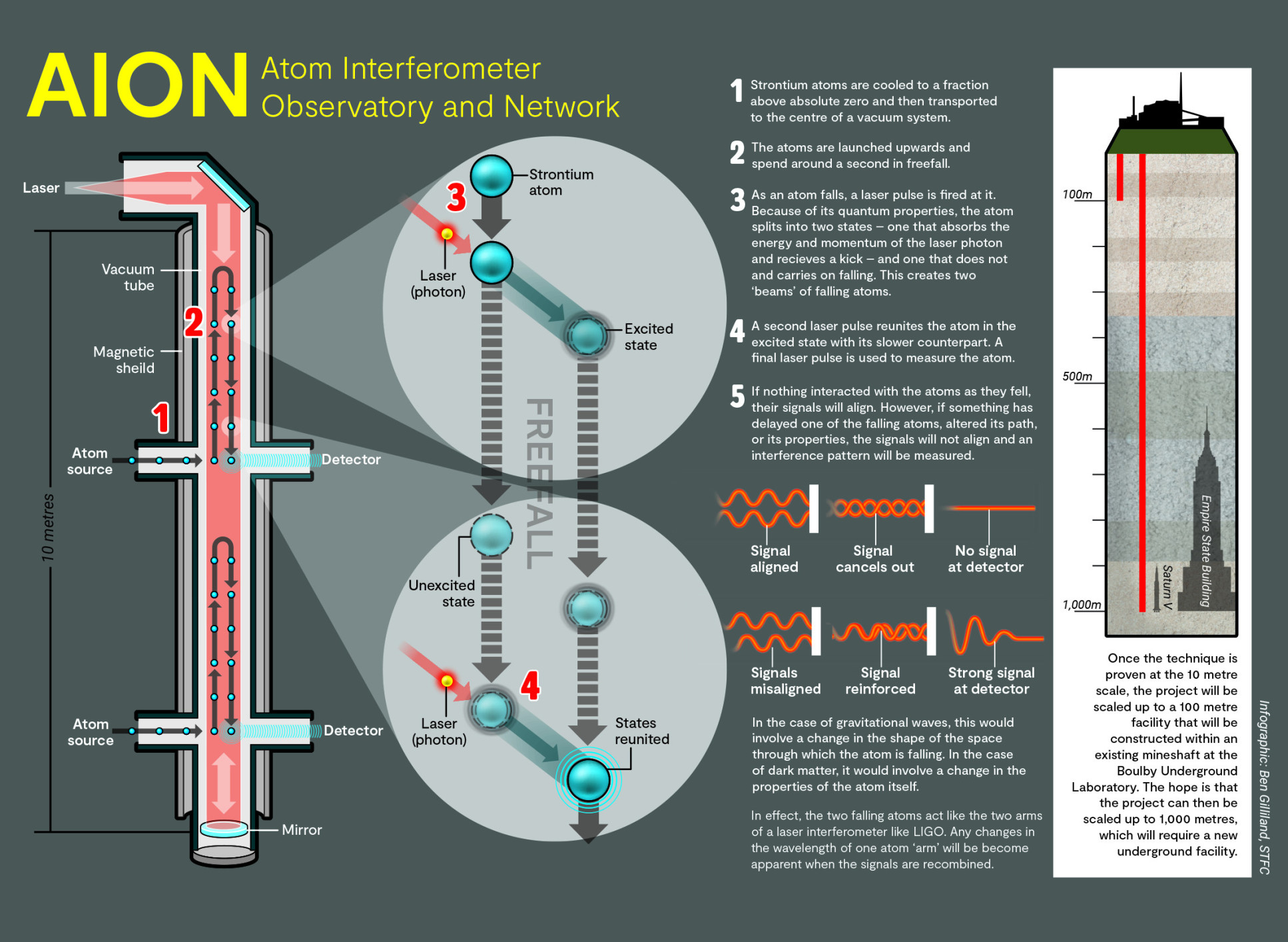
Here is an infographic explaining AION's experimental mechanism and long-term strategy. Image courtesy of Ben Gilliland, STFC
The AION collaboration hopes to combine their expertise and data with experiments conducting similar measurements, such as MAGIS-100 at Fermilab, to enhance the accuracy and reach of their measurements.
STFC Contributions
This intradisciplinary collaboration neccessitates a STFC cross departmental effort. The activities discussed here are closely intertwined and may be generalised for the sake of brevity.
The Finance staff within the Particle Physics Department manage the funding allocated by STFC to other project departments, including the Technology Department (TD) and RAL space . Additionally, the Particle Physics Department is responsible for modeling the guide field induced by magnetic coils and the layers of magnetic shielding surrounding the vacuum tube.
TD is currently working on the design of AION’s support structure, this building must contain the AION experimental apparatus within the restricted space of the Oxford Univeristy, Beecroft building.Their work aslo includes the design of interfaces that link this support structure and the experimental apparatus.
Image depicts a view 10m above a Placeholder for the AION interferometer in the Beecroft building at Oxford University.
The contributions of RAL Space to the project are many and varied. In 2022, RAL space worked with Imperial College to develop an optical glass cavity that would provide a stable reference for AION laser sources, ensuring they would deliver a consistent frequency to the Strontium atoms. RAL space have since applied this work to alternative laser frequency stabilisation techniques. Throughout 2024, members of RAL space conducted detailed measurements on Strontium atomic flux. Mimicing AION experimental apparatus; populations of Strontium atoms were cooled to milli Kelvin and collected via a magneto-optical trap allowing for measurements of their velocity and quantity.
Finally, the department is working to identify and mitigate potential noise sources that could affect the interferometer. In the coming year, highly sensitive instrumentation will be placed within a gravitationally intensive environment to detect and analyse these sources of noise. This research will ultimately inform on noise mitigation strategies for AION.
The Future of AION
AION-10 is the first iteration in a series of experiments, serving as a testbed for advancement of atom interferometery. The AION collaboration hopes to increase the baseline of its Atom Interferometer in successive experiments. Following this initial 10m experiment by 100m, then another experiment at the km scale. In the far future, >2050, the technology and principles could be applied to the AEDGE proposal, a space based atom interferometer.
Boulby Underground Laboratory is already being considered as a potential candidate for the site of AION-100 and AION-1000.